Power Quality And Voltage Flicker Explained
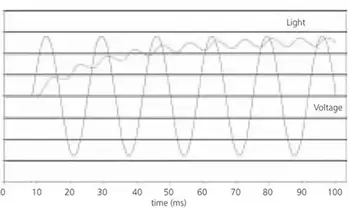
One of the many aspects of power quality that can affect electricity users is voltage fluctuation that causes lights to flicker. Whenever the electrical load changes, the supply voltage is affected proportionately. Most people have seen this occur in their house; when the refrigerator or the furnace starts, some of the lights may dim. If a large enough change occurs, such as the start-up of a large industrial motor, lights can dim or brighten, not only for that customer, but all over town. Normally the customer whose load is changing is the most affected, but other customers are all affected to some degree, depending on how large the load change is and how close they are to the changing load. If large voltage changes occur in rapid succession, light levels will vary, and when the variation becomes large enough to be noticeable or annoying, the effect is called light flicker. Experience has shown that most people are tolerant of an occasional dip in the lights, but when flicker is frequent or continuous, they begin to complain.
Power Quality Analysis Training
Request a Free Power Quality Training Quotation
HOW FLICKER IS PERCEIVED THE RESPONSE OF THE EYE
Like other human senses, the eye has amazing capabilities. Without conscious thought, our eyes can adapt to light levels varying by a factor of 10,000 from bright sunlight to faint starlight. Despite that enormous dynamic range, we can detect variations in brightness of less than 1%. Even more impressive, dedicated parts of the brain filter the incoming information, removing background clutter and extracting the most important features. Our eyes are also extraordinarily sensitive to rapid change. As the light level gradually drops by a factor of 100 at dusk, we may be almost unaware of the change, but a 1% step change in ambient light level due to a sudden change in voltage will almost certainly attract our attention. Fundamental constraints on the response of the eye limit our perception of very rapid events. The mechanisms of converting light into nerve impulses take a finite time to occur, so the brain has averaging mechanisms that smooth out the time delay between nerve impulses, presenting us with a constant picture, despite the “bucket brigade” nature of the incoming signals. As a result of this automatic smoothing, pictures displayed in rapid succession appear continuous, an effect that movies and television use to good advantage. Gaps shorter than about 50 milliseconds (1/20 of a second) are filled in, as our processing system ignores them as it would variations in nerve impulses, and averages them out. We are, therefore, quite insensitive to changes that occur at frequencies above about 20 cycles per second. It is no coincidence that our television and electric lights run at 60 cycles per second, fast enough that they do not appear to flicker. The net result of the balance between averaging and pupil adaptation is that our sensitivity to changing light levels increases the faster they change, up to the point where the finite response time of the eye and the automatic smoothing mechanism begin to come into effect. This begins to occur at about 9 cycles per second, the frequency at which we are most sensitive to light flicker.
The effect of voltage on light output There is one more level of complication involved when we consider the effect of variations in supply voltage. Since we are concerned with the effect of light flicker caused by voltage changes, we must consider not only the perception process, but also the conversion of electricity into light, and the way that the output level of a lamp depends on the power supply voltage. Different lamp types (incandescent, fluorescent, arc lamps) respond differently, but in general, the light output is proportional to the power consumption, although the power is not necessarily proportional to the voltage. In ballasted lamps, such as arc lamps and fluorescents, the ballast stabilizes the power, which is therefore more or less proportional to the voltage. Incandescent lamps act like resistors, so the power is roughly proportional to the square of the voltage (actually, the dependence is changed slightly by the fact that the resistance increases as the filament temperature increases). As a result, incandescent lamps tend to be the most sensitive to changing voltage, and so flicker calculations are based on incandescent lamps as a worst case. An incandescent lamp produces light by passing electric current through a tungsten filament until it glows white-hot. We tend to think that when we turn on the switch, the light comes on instantly but, in fact, it takes around a tenth of a second for the filament to heat up completely. Like any other object, it has a certain amount of thermal inertia, although because it is small, the time it takes to heat up is short. As we have seen, moderately fast changes are the most visible, and the filament time constant is close enough to the frequencies of interest that it must be taken into account. Thermal inertia provides another smoothing mechanism that reduces the impact of higher-frequency changes and slows down step changes. The thermal inertia depends on the size and shape of the filament, and so this term dictates a slightly different response curve in Europe, where 220-volt lamps are used, from North America, where the standard is 120 volts. You can see the light output of an incandescent lamp when the 60 Hz power is turned on. After the filament warms up, the light output has a substantial amount of flicker. Note that because the filament heats equally with positive and negative voltages, the flicker frequency is doubled to 120 Hz, too fast to be visible to the human eye.
From: Power Quality & UPS Handbook, Vol 11, The Electricity Forum
EF PARTNER MEDIA
Product Showcases
Shared Media