What is an Electrical Circuit?
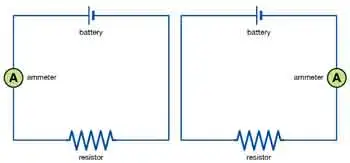
What is an Electrical Circuit?
An electrical circuit is a closed pathway for electric current flow, enabling diverse devices and systems to function. Therefore, gaining a grasp on the basics of electrical circuits, such as series and parallel configurations, voltage, current, resistance, Ohm's law, and circuit analysis techniques, is vital for anyone interested in the world of electronics, electrical engineering, or simply curious about the inner workings of everyday technology. By delving into these concepts, we can appreciate the underpinnings of countless technological advancements that shape our lives.
At its core, an electrical circuit is a closed loop or pathway that facilitates the flow of electric current. This concept is essential in electronics and electrical engineering, as it provides the basis for the operation of everyday items, including smartphones, computers, and home appliances. Within an electrical circuit, components are connected via conductive materials such as wires, enabling the movement of electrons from a power source to other components and back.
The primary components of an electrical circuit encompass a power source (e.g., a battery or power supply unit), conductive materials (typically wires), a load (like a resistor, motor, or light bulb), and a control element (a switch, for instance). The power source supplies the voltage necessary for electric current flow, while the load transforms electrical energy into other forms, such as light or heat. Meanwhile, the control element permits the user to initiate or halt the flow of electrons, effectively turning a device on or off.
Electrical circuits can be classified into three main types: series, parallel, and combination circuits. In a series circuit, components are connected end-to-end, with the current flowing through them sequentially. On the other hand, a parallel circuit features components linked to enable the current to follow multiple paths, distributing the current among different components. Finally, combination circuits comprise a mix of series and parallel configurations, resulting in greater complexity.
A fundamental understanding of voltage, current, and resistance is crucial for comprehending electrical circuit operations. Voltage, the driving force that propels electric charge through a circuit, and current, the flow of electric charge measured in amperes (A), are closely related to resistance. Resistance, expressed in ohms (Ω), represents the opposition to current flow. These elements are interconnected through Ohm's law, which posits that the voltage across a conductor is directly proportional to the current it carries and inversely proportional to the resistance: V = IR, where V signifies voltage, I denotes current, and R stands for resistance.
Circuit analysis determines the current, voltage, and power associated with each component in an electrical circuit. Techniques such as Kirchhoff's voltage and current laws, Thevenin's theorem, and Norton's theorem are employed to analyze and resolve electrical circuit issues. These methods enable engineers to design and troubleshoot electronic devices and systems effectively.
Circuit diagrams, or schematic diagrams, are graphical representations of electrical circuits that utilize standardized symbols to portray components like resistors, capacitors, inductors, diodes, and transistors. These symbols facilitate the interpretation of a circuit's structure and function by engineers or hobbyists without physically examining the actual components.
Thevenin's theorem
Thevenin's theorem is a fundamental principle in electrical engineering and circuit analysis. It is a powerful technique to simplify complex linear circuits, making it easier to analyze and calculate the current, voltage, and power across specific components. The theorem is named after the French engineer Charles Léonard Siméon Thévenin, who proposed it in 1883.
Thevenin's theorem states that any linear, active, bilateral network containing voltage sources, current sources, and resistors can be replaced by an equivalent circuit consisting of a single voltage source (called Thevenin's voltage, Vth) in series with a single resistor (called Thevenin's resistance, Rth) connected to the terminals of the original circuit. This simplified circuit, known as the Thevenin equivalent circuit, can then be used to analyze the behaviour of the original circuit with a specific load connected to its terminals.
To apply Thevenin's theorem and find the Thevenin equivalent circuit for a given network, follow these steps:
- Identify the portion of the circuit you want to simplify and the terminals where the load will be connected.
- Remove the load from the terminals (if present) and leave the terminals open-circuited.
- Calculate the open-circuit voltage across the terminals. This value is Thevenin's voltage (Vth).
- Calculate the equivalent resistance seen from the open-circuited terminals with all independent voltage sources replaced by short circuits (zero resistance) and all independent current sources replaced by open circuits (infinite resistance). This value is Thevenin's resistance (Rth).
- Create the Thevenin equivalent circuit with the calculated Vth and Rth values, connecting the original load across the terminals.
Once the Thevenin equivalent circuit is determined, you can easily analyze the circuit's behaviour and calculate the current through the load, the voltage across the load, or even the power delivered to the load. This technique is particularly useful when analyzing circuits with varying loads or examining the circuit's behaviour at multiple points, as it simplifies calculations and saves time.
Norton's theorem
Norton's theorem is an essential electrical engineering and circuit analysis principle that simplifies complex linear circuits for easier analysis. Named after the American engineer Edward Lawry Norton, who introduced it in the early 20th century, the theorem is a counterpart to Thevenin's theorem. While Thevenin's theorem reduces a complex network to an equivalent voltage source in series with a resistor, Norton's theorem simplifies the network to an equivalent current source parallel to a resistor.
Norton's theorem states that any linear, active, bilateral network containing voltage sources, current sources, and resistors can be replaced by an equivalent circuit consisting of a single current source (called Norton's current, IN) in parallel with a single resistor (called Norton's resistance, RN) connected to the terminals of the original circuit. This simplified circuit, known as the Norton equivalent circuit, enables the analysis of the original circuit with a specific load connected to its terminals.
To apply Norton's theorem and find the Norton equivalent circuit for a given network, follow these steps:
- Identify the portion of the circuit you want to simplify and the terminals where the load will be connected.
- Remove the load from the terminals (if present) and leave the terminals open-circuited.
- Calculate the short-circuit current flowing between the terminals. This value is Norton's current (IN).
- Calculate the equivalent resistance seen from the open-circuited terminals with all independent voltage sources replaced by short circuits (zero resistance) and all independent current sources replaced by open circuits (infinite resistance). This value is Norton's resistance (RN). Note that Norton's resistance is equal to Thevenin's, as both are calculated similarly.
- Create the Norton equivalent circuit with the calculated IN and RN values, connecting the original load across the terminals.
Once the Norton equivalent circuit is established, you can easily analyze the circuit's behaviour and calculate the current through the load, the voltage across the load, or even the power delivered to the load. Like Thevenin's theorem, Norton's theorem is particularly useful when dealing with varying loads or analyzing a circuit's behaviour at multiple points. In addition, it simplifies calculations, conserving time and effort.
What is an Electrical Circuit, and what are the common symbols used?
Circuit diagrams, or schematic diagrams, use standardized symbols to represent various electrical and electronic components. These symbols help engineers, technicians, and hobbyists understand a circuit's structure and functionality without physically examining the components. Here are some common symbols used in circuit diagrams:
Resistor: A simple zigzag line represents a resistor, which opposes the flow of electric current and dissipates energy in the form of heat.
Capacitor: Two parallel lines with a small gap represent a capacitor. The positive plate is marked with a "+" sign in polarized capacitors, and a curved line represents the negative plate.
Inductor: A series of curved or looped lines, similar to a coil, represents an inductor, which stores energy in a magnetic field and opposes changes in current.
Diode: A triangle pointing to a line represents a diode, which allows current to flow in one direction (from the triangle's point to the line) but blocks it in the opposite direction.
Light-emitting diode (LED): Similar to a diode symbol, but with two arrows pointing away from the triangle, representing light emission.
Transistor: Two types of transistors are commonly used: bipolar junction transistors (BJTs) and field-effect transistors (FETs). A BJT symbol comprises a circle or rectangle with three connected leads (emitter, base, and collector). FET symbols are represented by a combination of lines and a vertical arrow with three terminals (gate, source, and drain).
Integrated circuit (IC): A rectangular or square box with multiple leads connected represents an integrated circuit, a complex assembly of numerous electronic components within a single package.
Battery: Alternating long and short parallel lines represent a battery, a source of electrical energy.
Power supply: A circle with an arrow pointing upwards or a combination of letters, such as "Vcc" or "+V," represents a power supply, which provides a constant voltage or current.
Switch: A break in line with an angled line nearby or a pair of lines connected by a diagonal line represents a switch, which controls the flow of current by making or breaking a circuit.
Ground: A series of horizontal lines that decrease in length, a downward-pointing arrow, or the letters "GND" represent a ground connection, which serves as a reference point and provides a return path for electrical currents.
These are just a few examples of the many symbols used in circuit diagrams. Therefore, it's essential to familiarize yourself with these symbols to read or create schematic diagrams for electrical or electronic circuits.
What is an Electrical Circuit?
Electrical circuits form the foundation of modern technology, allowing us to harness the power of electricity to operate an array of devices and systems. This article delves into electrical circuits, discussing their key components, types, and characteristics while addressing some common questions related to the subject.